This is part one of a planned series on the environmental and resource impacts of direct air capture. Energy and water use are only part of the picture. In future installments, we will explore environmental impacts associated with CO2 pipeline and storage, DAC supply chains, and local environmental risks and benefits.
As of 2024, there are enough direct air capture (DAC) plants in the US to remove thousands of tons of CO2 per year. By 2030, this number could be much higher. Communities often question the resources used by DAC, especially energy and water consumption. This is an important question as more DAC facilities come online — even more so for host communities. This blog looks at the factors driving DAC’s direct energy and water consumption, the implications of DAC scale-up for energy and water availability in the short term, and ways to reduce potential strains on our energy and water systems.
Energy outlook for DAC
To estimate the amount of energy consumed by DAC in a year, we need to know two key parameters: (1) the energy required to remove a ton of CO2 and (2) the tons of CO2 removed per year. This relationship can be represented simply as:
Total EnergyDAC = (Energy per tCO2) * (Total DAC capacity)
For consistency, we’ll use units of kWH for energy and metric tons for capacity throughout. Let’s look at the first part: energy per tCO2. Estimates have put the energy required to remove one ton of CO2 between 1100kWH and 2500kWH. But the calculation isn’t so cut and dried; there are important nuances to these numbers to unpack first.
Energy per ton of CO2
DAC technology uses giant fans to move air into contact with materials that selectively capture CO2. Next, heat and pressure are applied to the capture materials to release CO2 for utilization or storage. Regeneration of the capture material is an energy intensive step that uses up over 70% to 90% of the energy consumed by DAC.
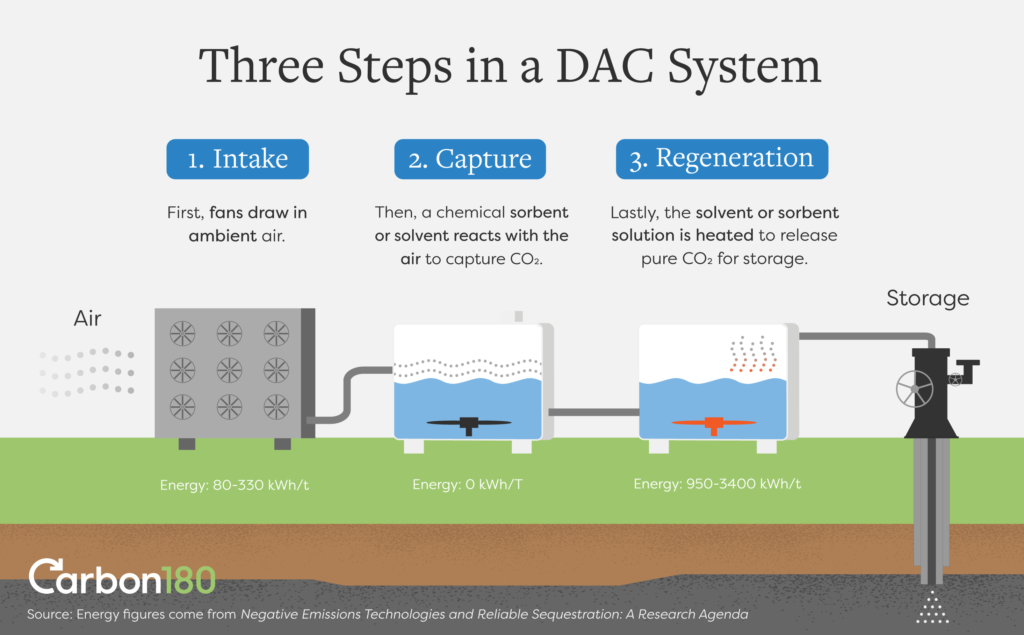
Crucially, the type of material used to capture CO2 is a key determinant of a DAC system’s energy consumption. Today, these materials fall into two major classes: solvents (used for liquid DAC) and sorbents (used for solid DAC). DAC solvents typically regenerate at around 900°C, which requires energy sources that can achieve high temperatures, such as natural gas or nuclear power. In contrast, the sorbents in solid DAC regenerate at around 100°C and can be powered by renewables or waste heat.
As a result, different energy mixes are needed to power liquid and solid DAC. Using numbers from commercial DAC systems available today, to remove one ton of CO2, liquid DAC systems need around 2550 kWh from sources like natural gas or nuclear to power materials regeneration alone (for step 3 in Figure 1), plus 205 kWh from solar or wind to power the remaining systems (for step 1). For solid DAC, around 1100 kWh is needed to power all three steps, all of which can come from renewable energy sources. To put this into perspective, an average home in the US uses about 900 kWh of electricity in a month. This difference in energy mixes is an important consideration as CDR scales up alongside the decarbonization of electricity production.
Total DAC capacity
Onto the second part of the equation: total DAC capacity. There are numerous projections of this in academic literature, but here we will focus on energy impacts from near-term deployments. Based on projects that are under construction or in development, the State of CDR report puts DAC capacity globally at 60Mt/year in 2030 – just over 1% of the amount of CO2 emitted by the US in 2023.. It is reasonable to assume this as a ceiling for US capacity, as not all projects will succeed or be based here.
Based on these estimates, powering DAC at 60Mt/year will occupy a minuscule portion of total US electricity production by 2030. If solid DAC is responsible for all 60Mt/year, DAC will use just over 1% of renewable electricity the US is projected to produce in 20301. However, if all DAC relies on liquid solvents, DAC will use more energy overall – about 0.5% of total projected electricity capacity, with most of the energy coming from nonrenewables. But because liquid DAC can’t use renewables to power the entire process, a smaller amount compared to solid DAC will come from renewables.
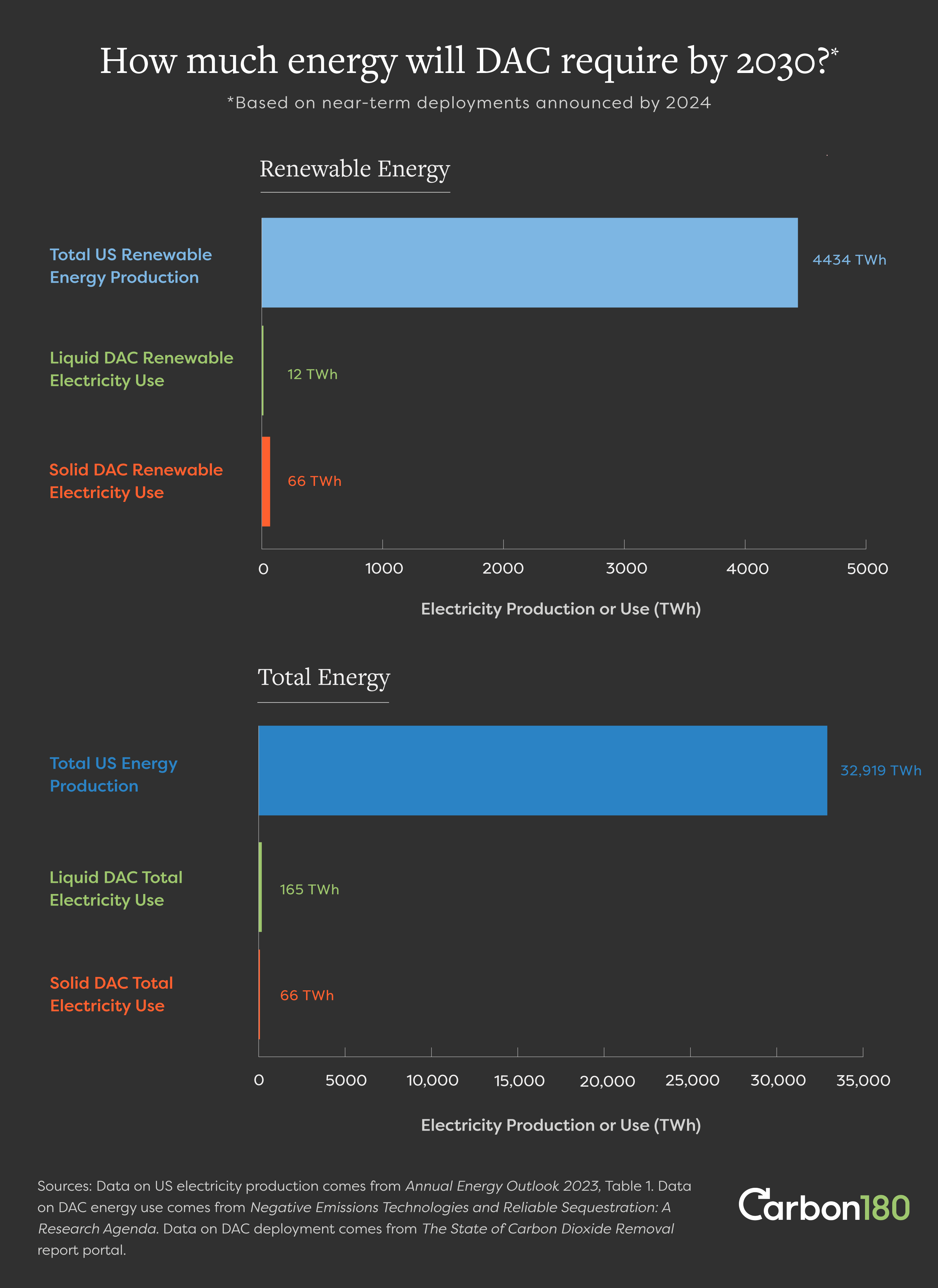
As we look to 2030, the overall impact on the US power grids from DAC will be very small. However, the actual energy impacts will likely be localized and acutely felt by communities that live near DAC facilities. The growth of data centers offers a useful comparison here. On the whole, data centers only take up 4% of US electricity. But in places with a high concentration of data centers, electricity consumption can be as much as 60%, leading to increased energy costs for local residents. Site selection for data centers depends on factors such as tax incentives and access to electricity and other infrastructure. DAC projects are similarly sited based on policy, proximity to geologic storage, and access to supporting infrastructure, as we are already seeing with the DOE DAC hubs. As DAC developers largely want to avoid competing for renewables meant for decarbonization, some developers are opting to build dedicated solar or wind capacity to power their DAC facilities, which may also alleviate the burdens on local electricity supply.
Outlook for DAC water use
Energy isn’t the only resource that DAC consumes. Liquid DAC consumes water for solvent regeneration, whereas solid DAC generally does not. In liquid DAC systems, the biggest source of water loss is evaporation during air contacting (the first step in Figure 1). As a result, water must be added to replenish the solvent. Just how much water is lost is highly dependent on the climate conditions at the DAC site. Under temperate conditions, liquid DAC systems are expected to consume 4.7 to 6.6 tons of water per ton of CO2 removed. In drier and hotter climates, more water is needed to counteract evaporation. Conversely, in wetter and colder climates, less water is needed.
Assuming a removal capacity of 60Mt/year in 2030, fully powered by liquid DAC systems, DAC will consume 282 million tons of water every year. This is equivalent to less than 5% of the water used by thermoelectric plants in the US in 2020, a small drop in the bucket of annual water use in the US.
As with energy impacts, DAC would likely exert highly localized impacts on water supply. Here too, data centers offer a helpful comparison, as they require huge quantities of drinking water to cool off servers and other equipment. While the amount of water used to cool data centers represents a small, though growing, portion of water consumption in the US, there can be major impacts at the local level. In The Dalles, Oregon, more than a quarter of the town’s annual water consumption goes toward cooling Google’s nearby data centers, leading to local concerns about water availability in a town that already sees drier seasons due to climate change. Similar challenges exist across the US, with research finding 20% of data centers are extracting water from stressed watersheds. DAC is coming online at a time when water availability is increasingly strained and when other commercial uses like data centers are competing for the same water resources.
Zooming out to the future
While the near-term impacts on electricity and water availability from DAC are small, if DAC were to reach gigaton capacity in 2050, it will have to scale by at least two orders of magnitude from the projected capacity in 2030. Using current estimates for DAC energy and water use, gigaton-scale DAC could significantly strain US energy and water systems by 2050.
Transparent data sharing by DAC developers will be important to shed light on energy and water consumption and to inform resource allocation. To pull one last lesson from the growth of data centers, less than a third of data center operators disclose their water consumption. This lack of transparency around water use obscures impacts on local water systems (link 1, link 2, link 3) and impedes long-term planning (link 1, link 2). For DAC, disclosure of energy and water consumption should be important components in a well-developed community benefits plan or similar agreements and publicly accessible.
Additional interventions could further limit DAC’s impact on resource use. First, the siting process for DAC facilities should take into account regional energy and water availability, to minimize impacts on local energy and water access. Second, we should continue research efforts into novel DAC materials and regeneration processes, which can make DAC more energy and water efficient. Third, DAC should be used judiciously — we should aim to deploy the minimum amount necessary, according to climate science and in concert with other climate mitigation strategies.
1. Includes grid-connected electricity from landfill gas; biogenic municipal waste; wind; photovoltaic and solar thermal sources; and non-electric energy from renewable sources, such as active and passive solar systems. Excludes hydroelectric and biomass energy source. (EIA Annual Energy Outlook 2023 Table. 1)
Edited by Tracy Yu. Image by American Public Power Association.